WHAT IS A STABLE TRANSFECTION?
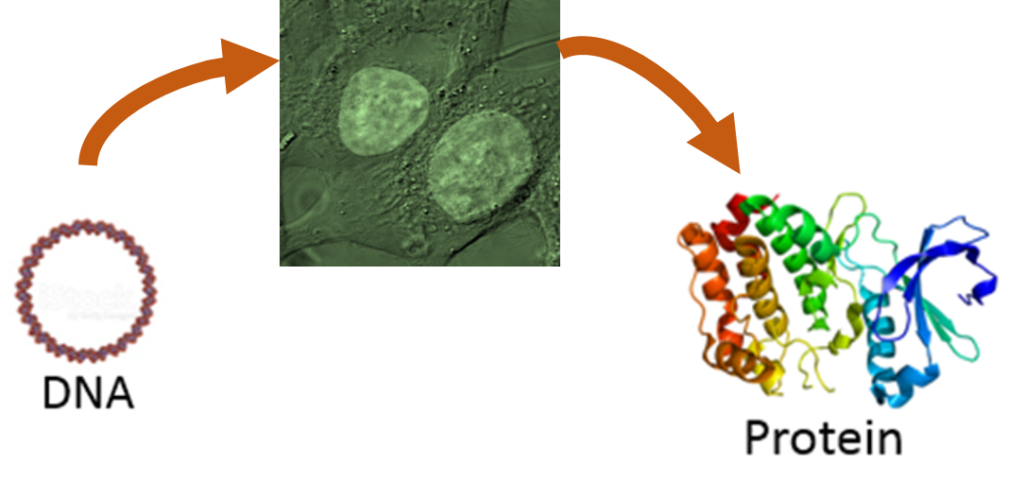
Transfection is a combination of the words “transfer” and “infection”. Transfection occurs when genetic material (i.e. DNA or RNA) “infects” a cell and is transferred into the cell’s genetic matrix.
Stable cell lines are created to overexpress a target protein in order to study gene functions, screen experimental drugs or produce therapeutic proteins. The newly created stable cell line indefinitely reproduces and continues to transcriptionally express the inserted gene.
This is a complex process that requires extreme patience and reliable equipment. Conveniently, there are professional labs that focus on developing stable cell lines. If a need arises for a specific type of transfected cell, there are many products and services at your disposal. Providing these services are cell line development companies called Contract Research Organizations (CROs).
WHAT IS CELL LINE DEVELOPMENT?
Cell Line Development is a method for which scientists create a large population of identical cells. It’s based on a simple idea that cells will divide by mitosis and continually produce genetically identical daughter cells until they reach senescence. Despite the simplicity of the theory, it is much more complex when a scientist wants a cell to express a gene that is not naturally expressed in that cell. This is an example where transfection can be utilized to insert genetic material into a specific cell.
HOW ARE STABLE CELL LINES DEVELOPED?
Immortalized cancer cell lines provide the innate transcription and translational machinery that enables stable cell line development. Three things are transferred into the cell. This is a relatively easy process helped by the cell’s natural ability to absorb plasmids in its environment. The three items are:
- Genetic Material (Gene of interest cloned into a DNA vector)
- Transfection Reagent
- DNA Encoding Antibiotic Resistance (Coded on the DNA vector backbone)
Briefly, the generation of stable cell lines is a two-step procedure. First of all, plasmid DNA is transfected into a host cell line. Consideration must be given to selecting the vector for which to insert the gene of interest, the transfection reagent ability to transfect plasmid DNA and the ability of the cell line to be transfected and express the integrated DNA. This step is then followed by selecting for positive clones by applying selective pressure using antibiotics. Only those cells that had successful integration of the plasmid DNA and are actively expressing the inserted gene can overcome the antibiotics since the backbone of the DNA vector contains an antibiotic resistance gene.
Generally, less than 5% of the genetic material makes its way inside of the cell membrane and then further to nucleus where it can integrate into the genomic DNA. Even then, it may never become a part of the host DNA. Integration is a very unlikely event, and there does not exist a good way of directly incorporating foreign genetic material into the genome.
The transfection reagent aids scientists in this process of successfully transfecting cells. A transfection reagent a tool used that helps the genetic material incorporate itself into the host DNA. The type of transfection reagent you use will depend on the type of host cell. There are a variety of different methods available, which can be tailored for your specific needs. Cell line specific and optimized protocols for transfection reagents can be purchased from vendors.
STABLE CELL SELECTION
If successful, roughly 5% of your transfected cells will contain your genetic alteration. Since it is not possible to physically pick them out one by one (they are far too small), an alternate route must be used to get rid of the negatively integrated cells.
This is the circumstance where the antibiotic resistance gene comes in to the picture. To create a “pure” cell culture containing the inserted gene, a solution that is toxic to the cells is introduced to the culture. This toxic solution eliminates the undesired cells that were not transfected with the plasmid. Theoretically, since successfully transfected cells should contain the genetic material for the target gene as well as an antibiotic resistance gene, the positively transfected cells are resistant to the antibiotic solution and survive the selective pressure.
STABLE CELL KILL CURVE
It should be noted that creation of a stably expressing cell line usually does not work as simply as just killing off untransfected cells and letting transfected cells live. The antibiotic usually will not be strong enough to kill a cell at low levels, a medium dose will simply slow cell growth and only a high dosage will kill cells. For these reasons, the dosage must be carefully calculated so that it will allow normal growth to continue for cells that have acquired the antibiotic resistance (i.e. the transfected DNA has integrated into the genome) but will cause the normal cells to significantly slow in growth and die. Over the course of a few weeks the normal cells will slowly disappear from the cell population; thus, leaving an entirely pure, positively integrated cell culture after approximately five weeks.
Cell sensitivity to an antibiotic varies between cell types. A kill curve tests the antibiotic resistance of a cell line by incorporating incremental steps in dose levels. This titration of antibiotic concentration helps to determine the minimal antibiotic concentration needed to kill the non-transfected cells. The kill curve should be determined prior to transfection of the stable cell lines.
Selective pressure on the cells by antibiotics typically starts 24-48 hours after transfection of the plasmid DNA. The following protocol provides general guidelines for determining the concentration of antibiotic needed to select mammalian cells:
- Plate the cells following standard procedures. Add concentrations of the antibiotic (i.e. antibiotic must match resistance gene in vector backbone) to the culture medium as a dilution series. All antibiotics have their own working range concentration.
- Incubate for 7 days and replace the antibiotic-containing medium every 4 days.
- At the end of 7 days, count viable cells in each well.
- Plot the viable cells versus the antibiotic concentration. This kill curve enables the researcher to determine the antibiotic concentration required to kill non-transfected cells. The optimal dose is determined as the lowest antibiotic concentration in which all cells are dead by day 7. Use the optimal dose concentration for stable cell line selection.